HBT interferometry is utilized in astronomy, quantum optics, and particle physics. HBT interferometry measures the size and spatial dispersion of a light source by comparing photon arrival times on two detectors as a function of their spatial separation.
A new HBT-based X-ray imaging technology can visualize the spatial arrangement of heavy elements in a crystal or molecule by forcing them to glow at X-ray wavelengths.
Now Fabian Trost of the German Electron Synchrotron (DESY) and colleagues—including some of those who first proposed the scheme—have implemented this technique and shown that the temporal correlation of fluorescence photons on a detector can image the structure of emitters on a copper film.
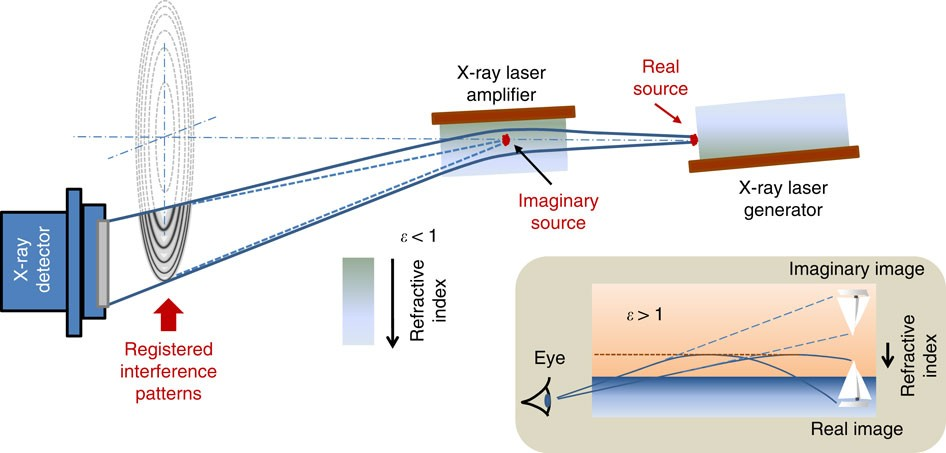
HBT interferometry now allows high-resolution x-ray imaging of biomolecules without crystallization.
The HBT effect happens when two indistinguishable photons from separate places in a source reach two detectors. When the two photons’ temporal interval is within the light source’s coherence period, they are indistinguishable.
The second-order correlation function, g(2), quantifies the interference impact, whether constructive or destructive. The interference fringes in g(2) will have less contrast if the arrival time exceeds the coherence time.
Due to the low likelihood of x-ray excitation and the short fluorescence coherence period, especially for heavy components, HBT has proved difficult to apply to x-rays. Fluorescence state duration determines coherence time.
The electronic state of a copper atom with a K shell vacancy lasts less than 1 fs. Copper atoms must be energized inside that interval to emit coherently. X-ray free-electron lasers (XFELs) enable the creation of high-intensity x-ray pulses of femtosecond or shorter durations. These pulses can ignite heavy element K-shell electrons and produce indistinguishable photon pairs by x-ray fluorescence.
Trost and colleagues measured the intensity correlation of copper screen fluorescence photons using such pulses. The researchers utilized a phase grating to focus the incoming x-ray pulse on two points on a micron-sized copper foil at the European XFEL facility in Germany.
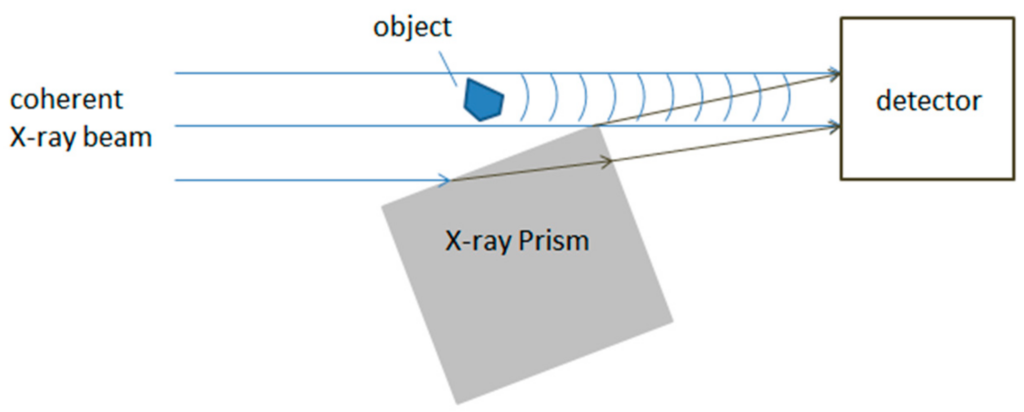
The experimental realization of a recently proposed approach suggests novel x-ray molecular imaging potential.
The film’s lit copper atoms’ K-shell electrons were ionized by 9 keV x-ray photons. The XFEL detector monitored fluorescence emission from a short-lived excited state formed by this ionization process. This detector measures 1012 correlations between pixel pairs with one million single-photon-detecting pixels.
This technology required the team to overcome various obstacles to adopt x-ray imaging. The detector employed in the experiment cannot resolve photon energy, therefore it cannot eliminate radiation from sources other than Kα emission. Nonselectivity lowers signal-to-noise ratio. Trost and colleagues blocked elastically dispersed and copper Kβ rays with a nickel filter.
The interference fringes were less visible because the x-ray pulse was 10 times longer than the Kα emission’s coherence lifetime. The detector’s fast readout rate and XFEL pulse repetition rate allowed the researchers to record 58 million 2D fluorescence images in around 5 hours to improve signal quality.
The copper foil was rotated so each pulse lighted a fresh location to protect the sample. The combined fluorescence image was isotropic and had no structural information, but the produced g(2) showed interference fringes reaching the third-order peaks.
Researchers iteratively constructed the copper film’s two stimulated spots’ size (300 nm) and separation (860 nm).
With enough spatial resolution, the approach may image biomolecules and catalysts at the atomic scale and characterize their reaction kinetics. XFEL technology may provide fluorescence excitation with subfemtosecond pulses. Using strong, subfemtosecond x-ray pulses, the temporal fluorescence profile can be shorter than the lifetime of the fluorescing states.
Multicolor imaging with multiple detectors or energy-discrimination detectors is needed to take use of HBT interferometry for chemical imaging with elemental specificity.
This could revolutionize the characterisation of essential catalytic functions and structural alterations in their native settings, such as metal-bearing clusters in metalloproteins [9]. The development of renewable energy sources requires extraordinary insight into these catalysts’ structure and function.